|
 |
VESTIBULAR SYSTEM
The
vestibulocochlear nerve (VIII) has the dual function of serving
both the sense of hearing (via cochlear fibers) and
proprioception (via vestibular fibers).
THE
VESTIBULAR APPARATUS
A cavernous
network called the bony labyrinth exists within the temporal
bone on either side of the head. Within this bony labyrinth is a
membranous labyrinth of roughly the same shape filled with
endolymph, the same fluid present in the cochlear duct of the
inner ear (Fig-1). The endolymph in both the vestibular and
cochlear systems is continuous, and is formed in the endolymphatic sac, which makes contact with the fluid of the
temporal dura. The space between the membranous and bony
labyrinths is filled with perilymph. The
membranous labyrinth is composed of three semicircular canals.
Each canal
is twice connected to the utriculus, a large endolymph-containing
sac. The endolymph of each canal is continuous with that in the utriculus at one end, and
separated from it at the other end by a flexible
mechanosensitive barrier called the crista ampullaris. The
crista is located in the enlarged end of each canal known as the
ampulla. The anterior and posterior canals are essentially
vertical when a person holds his head erect and they are at
right angles to each other. The lateral canal is almost
horizontal (actually elevated 23° anteriorly) and forms a plane
at right angles to the other two. This geometric arrangement
provides the vestibular system with the capability of detecting
movements of the head in all directions.
The
utriculus is continuous with a second endolymphatic enlargement,
the sacculus. A mechanosensitive structure, the macula acustica,
is located in the wall of the utriculus with a second macula
located in the saccular wall. The three cristae and two maculae
are the actual proprioceptive units in each vestibular
apparatus. The cristae and maculae are in neural contact with
the central nervous system through SSA VIII nerve fibers.
Mechanosensitive hair cells in the cristae and maculae form
two-element receptors with these fibers.
|
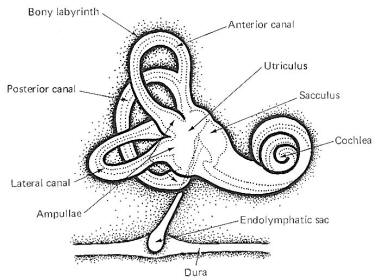 |
Fig-1 |
Figure-2
illustrates the distribution of the vestibular nerve fibers to
the membranous labyrinth. Notice that one branch of the nerve is
distributed to each ampulla, where it distributes to the crista
ampullaris hair cells. Separate branches of
the nerve are also distributed to the maculae of the utriculus
and sacculus, where they form two-element receptors with the
macular hair cells.
The Crista
Ampullaris
The crista
ampullaris is a mechanosensitive flexible barrier to the flow of
endolymph between one end of the semicircular canal and the
utriculus (Fig-3). A number of sensitive hair cells are
interposed with supporting cells at the base of
the crista within the ampulla. The hair cell hairs project into
a gelatinous mass, the cupola, which projects upward to form a
flexible barrier across the space of the ampulla. The cupola behaves like an elastic
diaphragm rather than like a swinging door. Angular movements of the head cause the endolymph to
push against the cupola so that it bows in one direction or the
other. Deflection toward the utriculus is utriculopetal
deflection, while deflection away from the utriculus is
utriculofugal deflection. Deflecting the cupola bends the hairs,
excites the hair cells, and produces impulses in the SSA VIII
nerve fibers. In this way the CNS is informed of movements of
the head.
|
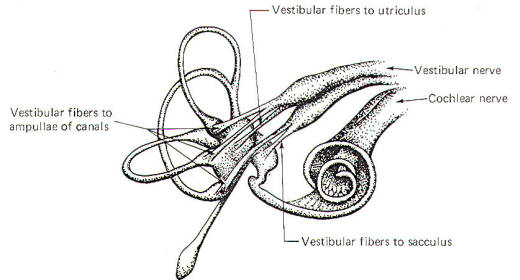 |
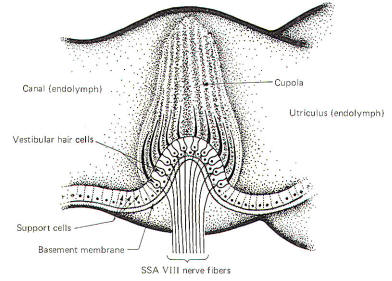 |
Fig-2 |
Fig-3 |
There are
two types of hair cells in the vestibular apparatus. Type I hair
cells are somewhat spherical in shape with 60 to 70 small hairs
(stereocilia) emerging from the cuticle (Fig-4). A
particularly long hair process, the kinocilium, stands at one
end of the stereocilia. Type II hair cells are more cylindrical
in shape but their stereocilia and kinocilia are identical with
type I cells.
SSA VIII
nerve fibers are in close contact with both types of cells,
although they form more extensive processes around the base of
type I cells. In addition to the SSA fibers, there is evidence
that small-diameter efferent fibers of unknown origin also
innervate the hair cells. They form direct synaptic contacts
with the type II cells but appear instead to terminate on SSA
fibers of the type I cells. The origin and function of these
efferent fibers is unknown. It seems likely that they may in
some way influence the excitability of the hair cells and their
potential for producing impulses in the SSA VIII nerve fibers.
Hair Cell
Stimulation and Cochlear Nerve Discharge
The
stereocilia and kinocilium of each hair cell project up into the
gelatinous cupola. Consequently, whenever the cupola is
displaced, either toward the utriculus or away from it, the
hairs are also deflected. Deflection of the hairs toward the
kinocilium produces a change in the hair cell sufficient to
increase the firing rate in the SSA VIII nerve fibers.
Conversely, deflection away from the kinocilium decreases the
firing rate (Fig-5).
|
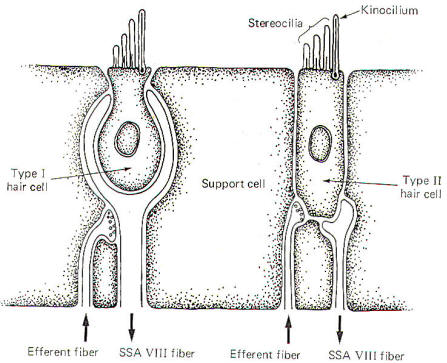 |
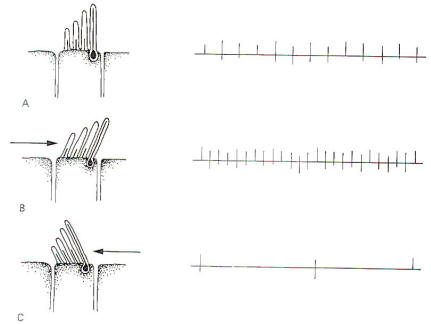 |
Fig-4 |
Fig-5 |
The hair
cells in a given crista ampullaris are all orientated in the
same direction so that deflection of the cupola either bends
all the hairs toward the kinocilia or away from it. Thus
deflection of the cupola either increases or decreases the
firing rate of the SSA VIII nerve fibers.
In the
lateral canals, the kinocilia all face the utriculus. In the
vertical canals they all face away from the utriculus, toward
the canal. Thus, utriculopetal deflection in the lateral canals
produces an increase in the firing rate, while utriculofugal
deflection produces a decrease. However, just the opposite is
true concerning the vertical canals. Here the hair cell
kinocilia are oriented in the opposite direction so that
utriculopetal deflection causes a decrease while utriculofugal
deflection produces an increase in the firing rate.
Coplanar
Canals are Functional Units The anterior canal on one side of
the head and the posterior canal on the opposite side are in the
same plane. Thus the two canals are a functional unit since any
head movement which causes utriculofugal deflection in the
anterior canal on one side will be matched by utriculopetal
deflection in the posterior canal on the opposite side (Fig-6). A similar relationship exists with the two lateral canals
and they also form a functional unit (Fig-7).
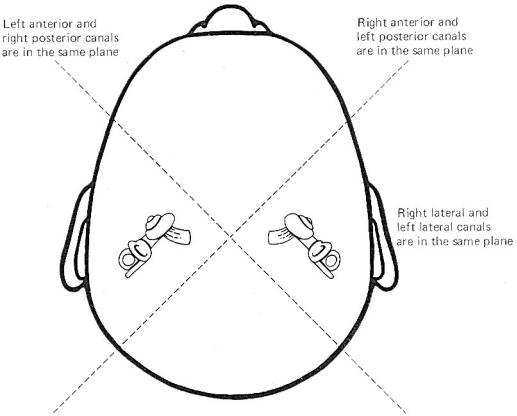 |
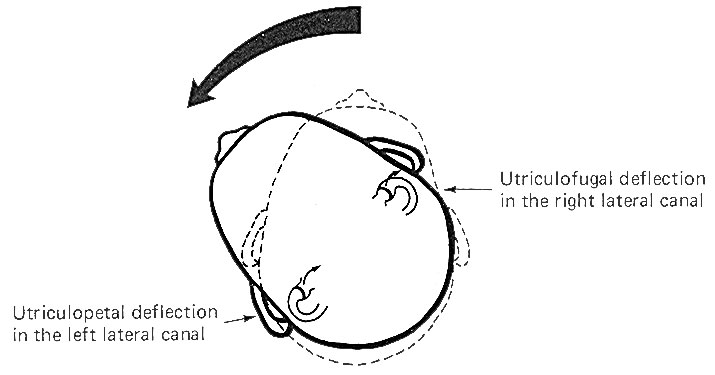 |
Fig-6 |
Fig-7 |
Hair cells stimulate SSA VIII nerve fibers via
chemical synapses. Because a fairly steady resting discharge of
40 to 60 impulses per second can be recorded in the nerve
fibers, it is assumed that a small amount of transmitter
chemical (possibly a catecholamine) is constantly being
released. It has been proposed that displacement of the hairs
toward the kinocilium increases the firing rate by increasing
the rate or amount of transmitter released by the hair cell.
Likewise, displacement of the hairs in the opposite direction
decreases the firing rate by lowering the rate or amount of
release.
In
contrast to the stereocilia, which are embedded in the cuticle,
the base of the kinocilium is in direct contact with the hair
cell cytoplasm. The kinocilium plunging inward (with the aid of
the stereocilia leaning against it) may depolarize the hair cell
membrane and establish a receptor potential, which in turn
causes transmitter release. Alternatively, deflection of the
stereocilia away from the kinocilium pulls the kinocilium
outward, hyperpolarizing the membrane and decreasing
transmitter release.
The cristae
are particularly sensitive to changes in angular acceleration
and deceleration of the head. The greatest change in firing rate
along nerve fibers from the cristae occur at the beginning and
end of angular movements of the head. As Fig-7 shows, the
inertia of the endolymph when the head first starts rotating to
the left produces utriculopetal deflection in the left canal and
utriculofugal deflection in the right canal. Thus we see a large
initial change in firing rate from each canal at the beginning
of the movement. However, if the rotation of the head to the
left continues, we see no further change in firing rates until
the rotation begins to slow down (decelerate). At this point.
the inertia of the endolymph causes the cupola to deflect in the
opposite direction, once again causing a change in the firing
rate. This time, however, there is a decrease in the left canal
and an increase in the right canal. Thus one can see that the
canal system is particularly adept at signaling changes in
acceleration and deceleration of the head's angular movements.
Further, because the canals are arranged in three planes,
angular movements in all directions are easily detected by the
canal system. No doubt angular movements which are not exactly
parallel with a single coplanar canal system are detected by the
brain through some "weighted" input from two or more coplanar
functional units.
The Macula
Acustica
The macula
acustica is a mechanosensitive structure in the utriculus and
sacculus. It is similar to the crista in that the base of the
structure is composed of type I and type II hair cells (Fig-8). Likewise, the base of the hair cells
forms contacts with
SSA VIII nerve fibers. Maculae are also called otolith receptors
because the hair processes project into a low-lying gelatinous
structure which is impregnated with dense calcareous formations
called otoliths or otoconia. The otolith receptors respond to
static gravitational pull and are therefore well equipped to
signal the position of the head in space at any given time. A
basal discharge rate of the SSA VIII fibers from the utriculus
is observed when the head is in the normal erect position. This
rate increases to a maximum
when the head is moved to a position 90° from normal (i.e., 90°
forward, backward, or to either side).
In addition
to their gravitational or static response, utricular otolith
receptors also respond to linear acceleration and deceleration
of the head, thus exhibiting a dynamic response characteristic
as well. Saccular otolith receptors respond only to the static
position of the head in space and demonstrate no appreciable
dynamic response. |
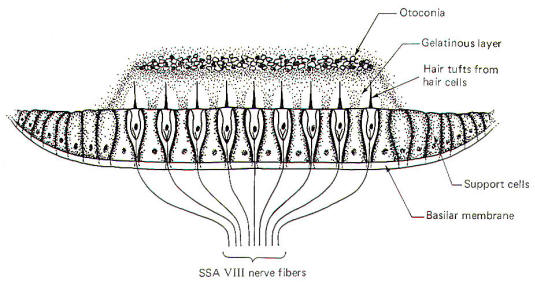 |
|
Fig-8 |
VESTIBULAR
SYSTEM INTERACTIONS
Vestibular
Control of Eye Movements
An
interesting cooperative relationship exists between the
vestibular system and the extraocular muscles of the eye. Those
eye movements caused by vestibular stimulation are generally
compensatory in nature, attempting to keep to the visual axis
relatively fixed when the head is moved in space. This aids both
vision and the maintenance of posture. As an example, a
cooperative relationship exists between the lateral canals on
both sides of the head that is designed to keep the eyes
directed toward a reference point in the visual field as the
head is moved in a lateral plane (Fig-9). Unless consciously
overridden, the eyes move slowly to the left as the head is
turned slowly to the right, maintaining a constant reference
point in the visual field.
These
reflex conjugate eye movements are produced by changes in
activity of the extraocular eye muscles in response to
vestibular activity. A close examination of Fig-9 shows
that when the head is turned to the right, the endolymph in the
right lateral canal deflects the cupola toward the utriculus (utriculopetal),
while the endolymph of the left lateral canal deflects its
cupola away from the utriculus (utriculofugal). Now if we
remember that utriculopetal deflection in the lateral canals
increases the firing rate while utriculofugal deflection
decreases it, an examination of the neural circuitry in Fig-9 explains the slow shift of the eyes to the left. The
lateral rectus muscle of the left eye and medial rectus of the
right eye both contract, while their antagonists relax, pulling
the eyes slowly to the left. A similar cooperative relationship
exists between the anterior canal on one side and the posterior
canal on the other. The anterior canals are able to produce
stimulation of the ipsilateral superior rectus muscle and the
contralateral inferior oblique. The posterior canals produce
stimulation of the ipsilateral superior oblique and the
contralateral inferior rectus muscle. In this way the eyes can
maintain their reference point when the head is moved through
any plane.
The
Vestibulospinal System
While the
vestibular system responds primarily to movements of the head,
it is able to produce far-reaching postural changes throughout
the body. The vestibular system can regulate alpha and gamma
motor neuron activity in the spinal cord through the lateral
and medial vestibulospinal tracts (Fig-7). The
vestibulospinal tracts originate in the vestibular nuclei of the
brainstem. Those fibers which originate in the lateral
vestibular (Deiter's) nucleus descend ipsilaterally in the
anterior funiculus and form the lateral vestibulospinal tract.
The fibers of this tract terminate in laminae VII, VIII, and IX
at all levels of the cord. Arising from the medial vestibular
nucleus are the fibers of the medial vestibulospinal tract.
While there is a small crossed component, most of its fibers
descend ipsilaterally only as far as the midthoracic level,
where they too synapse in laminae VII, VIII, and IX.
The
vestibulospinal tracts facilitate extensor and inhibit flexor
alpha and gamma motor neurons. Input to the vestibular nuclei
via fibers of cranial nerve VIII from the vestibular apparatus
presupposes an antigravity or postural role for the
vestibulospinal tracts. Activity in these tracts is also
influenced by input to the vestibular nuclei from the
cerebellum, and through it, the peripheral proprioceptors of
muscles, tendons, and joints.
|
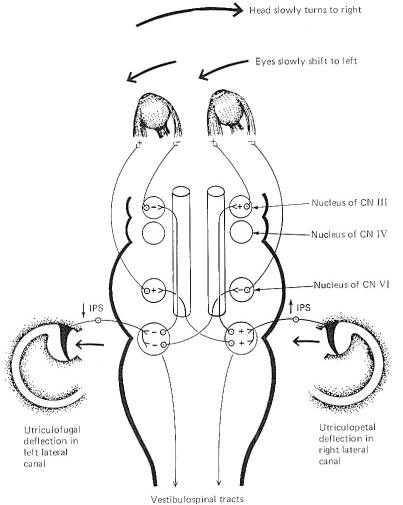 |
Fig-9 |
The
Vestibular System and the Cerebellum
Because of
the role the vestibular system plays in the maintenance of
posture and muscle control, it is not surprising to find that
the system has a close relationship with the cerebellum. Both
first- and second-order vestibulocerebellar fibers end as mossy
fibers on the granular cells of the cerebellar cortex of the
flocculonodular lobe. In addition, the fastigial and dentate
cerebellar nuclei also receive vestibular input. Presumably the
cerebellar cortex integrates the vestibular input with other
proprioceptive input from all parts of the body. The cerebellum
is then in a position to exert influence on the postural
musculature via output to the vestibular, reticular, and red
nuclei. Vestibulospinal, reticulospinal, and rubrospinal fibers
influence muscle activity at the spinal cord level, while
cerebellar output through the thalamus to the cerebral cortex
modifies motor activity at the cortical source.
Vestibulocortical Projections
In order to
be consciously aware of position and movements of the head in
space, it is necessary that vestibular information reach the
cerebral cortex. The kinesthetic sense (conscious awareness of
body position and movement) requires cortical input from
peripheral proprioceptors as well as from the vestibular
system. The cortical area which receives this information is
located in the postcentral gyrus near the somatosensory
projection of the mouth. Vestibulocortical projections appear to
be primarily contralateral with intermediate synapses in the
ipsilateral vestibular nuclei and the contralateral thalamus.
Vestibular
System and Autonomic Effects
The effects
of vestibular activity on autonomic function are well known and
are grouped under the heading "motion sickness." They include
effects on the vasomotor system (typically a vasodepressor
action with a blood pressure drop), an increase in the rate and
depth of respiration, decreased salivation, increased sweating,
pupillary dilation, and disturbances of the gastrointestinal
tract. Most of these effects are mediated through the
sympathetic nervous system.
Tests for
the Integrity of the Semicircular Canals
Certain
bodily responses to vestibular stimulation are reflexly
predictable, such as conjugate movements of the eyes and other
postural adjustments of the body. The integrity of the various
canals can be tested by their capacity to produce the expected
responses. The rotation (swivel chair) test and the caloric test
are both designed to do this.
The
rotation test allows maximum stimulation of the horizontal and
vertical canals. Maximum deflection of the cupola of a
particular canal occurs when the movement of the head is in the
same plane as the canal which contains that cupola. This is
accomplished in the swivel chair by placing the head in various
positions and then rotating the chair. Recall that maximum
deflection in a canal on one side of the head is accompanied by
maximum deflection in its functional counterpart on the opposite
side.
Predictable
responses observed with rotation tests are nystagmus, vertigo,
and past pointing. Nystagmus refers to rapid to-and-fro movements
of the eyes. As previously noted, the eyes slowly shift to the
left as the head is turned slowly to the right. Of course there
is a limit to how far left the eyes can shift if the head
continues turning to the right. When they have pulled as far
left as possible, they suddenly "snap" back to the right and
"fix" on a new reference point in the visual field. This
alternating slow phase to the left followed by a fast phase to
the right continues as the head keeps rotating to the right
unless consciously overridden. While nystagmus technically
refers to the eye shifts in both directions, neuroscientists
typically refer to nystagmus as the direction of the fast phase.
For example, nystagmus is to the right in the case just
described.
Because
cupola deflection directly controls eye movements, and because
this deflection is in one direction during the acceleration
phase of the angular rotation and in the opposite direction
during the deceleration phase, it follows that nystagmus is in
one direction during rotation (perrotation) and in the opposite
direction after rotation (postrotation). Perrotational nystagmus
is in the same direction as the rotation. However, if the
rotating chair is suddenly stopped, the canals cease to rotate
but the inertia of the endolymph is not so easily overcome.
Consequently the cupolae are deflected in the opposite direction
for a brief period of time, producing a postrotational nystagmus
in the direction opposite the rotation.
Vertigo and
past pointing are also predictably observed following rotation
in a normal individual. Vertigo is the sensation of a movement
when no such movement exists. This is caused by the fact that
once the actual turning stops, the inertia of the endolymph
remains for a while, deflecting the cupolae and sending signals
to the brain that turning is still occurring. Normally the
vertigo (false sense of movement) is in the same direction as
the postrotational nystagmus. The body will ordinarily attempt
to reflexly make postural adjustments for the vertigo just as it
would for a real movement. Thus, predictable leaning of the
whole body (a reflex attempt to correct for the false movement)
is typically observed following a period of rotation.
Specifically, the body leans in the direction opposite the postrotational
nystagmus, An
extended arm also points in the direction opposite the post
rotational nystagmus. This is past pointing,
The
rotation test has the disadvantage of not allowing the canals on
each side of the head to be tested separately. However, caloric
tests, which involve the introduction of hot or cold solutions
into the auditory canal, allow the clinician to test each side
of the head separately. A hot water solution introduced into the
auditory canal causes the endolymph to expand, deflecting the
cupola in a predictable direction. This is later followed by the
use of a cold water solution which cools the endolymph,
producing deflection in the opposite direction. Like the
rotation test, predictable changes in nystagmus, vertigo, and
past pointing can be observed.
|
 |
 |
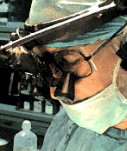
Prof. Munir Elias
Our brain is a mystery and to understand it, you
need to be a neurosurgeon, neuroanatomist and neurophysiologist.
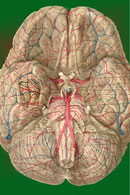
neurosurgery.tv

Please visit this site, where daily neurosurgical activities are going
on.
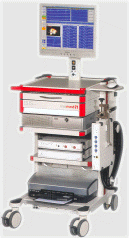
Inomed ISIS IOM System
|
|
|
|