THE HYPOTHALAMUS
The hypothalamus
forms the floor of the third ventricle and is separated from the
thalamus above by the hypothalamic sulcus in the ventricle's
lateral walls. It is composed of a discrete set of nuclei (Fig-1
and 2) which are involved in the following functions:
1 Autonomic control
2 Temperature
regulation
3 Thirst and
control of body water
4 Appetite control
5
Endocrine control
6
Emotional reactions
7 Sleep
and wakefulness
8 Stress response
Hypothalamic Nuclei
Several
nuclei have been identified in the hypothalamus.
Some have become associated with specific
physiological activities, while the functions of
others are less clear and in some cases unknown.
Their relative locations are illustrated in
midsagittal section in Fig-1 and 2. Therefore it is
important to recognize that you are seeing the
nuclei on the right side of the third ventricle
only. In other words, each of
the nuclei is paired. The
nuclei are often grouped in four general areas. The
preoptic
area includes the medial and lateral preoptic nuclei,
which extend through the lamina terminalis. The
supraoptic area
includes the supraoptic, anterior hypothalamic, and paraventricular nuclei. The tuberal
area include
the lateral hypothalamic, posterior hypothalamic, dorsomedial, and ventromedial nuclei. Finally, the
mammillary area is
composed of the medial and lateral mammillary
nuclei.
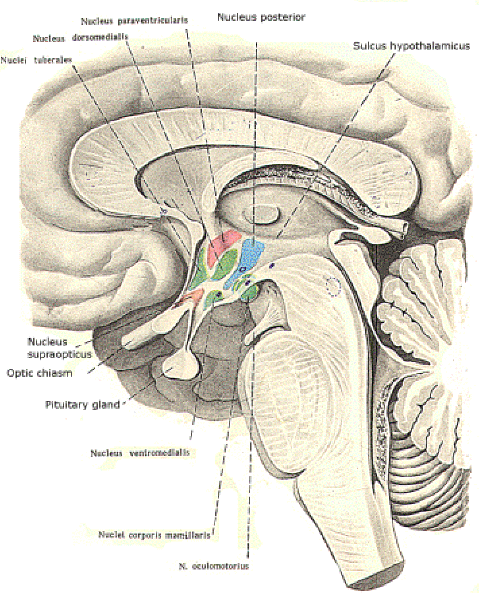 |
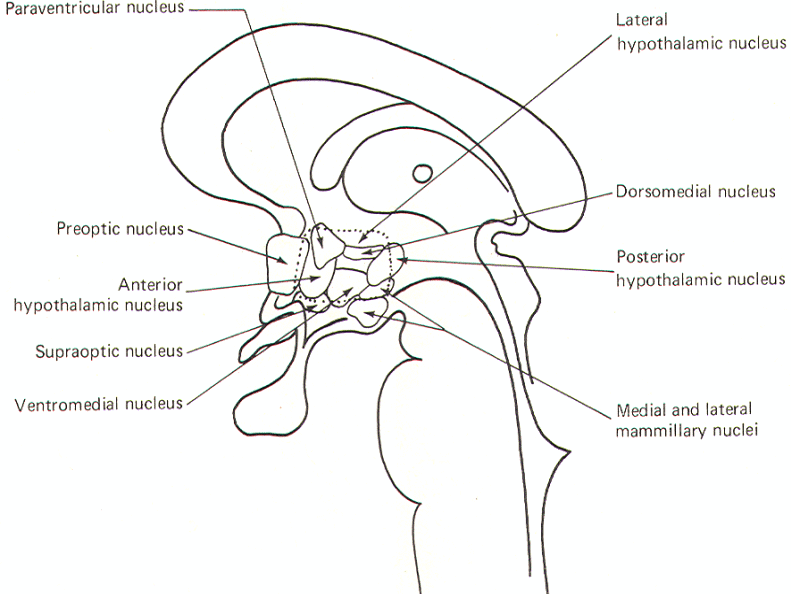 |
Fig-1: |
Fig-2: |
Hypothalamic Connections
For the
hypothalamus to play an effective role in the
functions listed above, it is necessary that it be
in neural contact with many areas of the brain and
spinal
cord.
The fiber systems involved can be described as
either afferent or efferent to the hypothalamus.
Some of the principal systems are presented below.
Hypothalamic Afferent
Input
Fibers
in the mammillary peduncle represent a major
ascending input to the hypothalamus (Fig-3). It
arises in the tegmentum of the midbrain and is
formed by fibers carrying information from SVA and GVA fibers which terminate in the solitary
nucleus. Similarly, ascending information from the
spinal cord relayed through the medial lemniscus
also contributes fibers to this system. The
hypothalamic termination is chiefly in the lateral
mammillary nuclei.
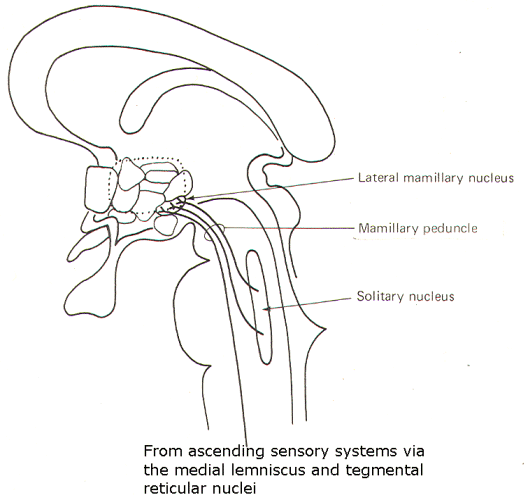 |
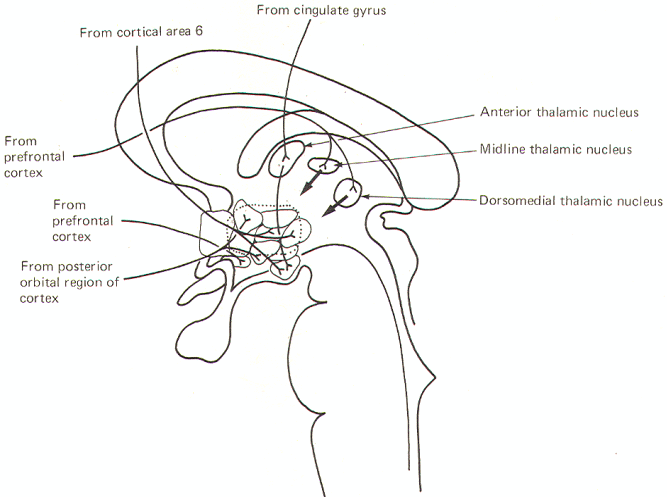 |
Fig-3 |
Fig-4 |
The
corticohypothalamic
fibers
project
to a number of hypothalamic nuclei. It is no doubt
through such connections that conscious thought is
often able to give rise to autonomic and visceral
responses such as, for example, indigestion from
worry, sweating from fear, and sexual arousal from
certain kinds of thoughts. Nevertheless, the
hypothalamus is not ordinarily under cortical
control as evidenced, for example, by our inability
to raise or lower the blood pressure at will.
Several corticohypothalamic routes are illustrated in Fig-4.
Fibers from
cortical area 6 pass through the septal region to
terminate chiefly in the posterior hypothalamic and
lateral hypothalamic nuclei as well as the
mammillary nuclei. Fibers from the prefrontal
cortex project to the supraoptic nucleus as well as
indirectly to the hypothalamus through synapses in
the anterior, midline, and dorsomedial thalamic
nuclei. Projections from the olfactory posterior
orbital region of the cortex project to the
paraventricular and ventromedial nuclei. The
cingulate gyrus also indirectly influences the
hypothalamus via an intermediate synapse in the
anterior thalamic nucleus. Thalamomammillary fibers
are also present.
The
thalamohypothalamic fibers
fall
into two general groups; the thalamomammillary
fibers which project from the anterior thalamic
nucleus to the medial mammillary nucleus, and a
group which passes from the midline and dorsomedial
thalamic nuclei principally to the anterior
hypothalamic nucleus. There are probably other
connections as well between the thalamus and
hypothalamus (Fig-5).
The
corticomammillary fibers
(fornix) project from the hippocampus of the temporal lobe to
the mammillary nuclei via a long loop (Fig-6). The
stria terminalis
is composed of fibers which originate in the amygdala of the
temporal lobe and pass caudally along the tail of the caudate
nucleus and arch over the dorsal aspect of the thalamus to
terminate in the septal nuclei as well as the preoptic, anterior
hypothalamic, and ventromedial nuclei. The
medial forebrain bundle is a complex group of
fibers which arise in the basal olfactory region,
the septal nuclei, and periamygdaloid region and
pass to the lateral hypothalamic nuclear area (Fig-7). Many medial forebrain bundle fibers continue
into the midbrain tegmentum while others project to
additional hypothalamic nuclei. Those reaching the
midbrain tegmentum relay signals to the autonomic
and visceral controlling nuclei of the brainstem.
Hence the bundle is both an afferent and efferent
system with respect to hypothalamic nuclei.
Hypothalamic Efferent Output
The anterior thalamic
and mammillary nuclei are reciprocally related and
therefore a mammillothalamic tract exists.
Through projection fibers from the anterior thalamic
nucleus to the cingulate gyrus, the hypothalamus is
able to influence activity in this region of the
cerebral cortex. This system and the mammillotegmental fibers which project to the
reticular nuclei of the brain stem tegmentum are
illustrated in Figure-8.
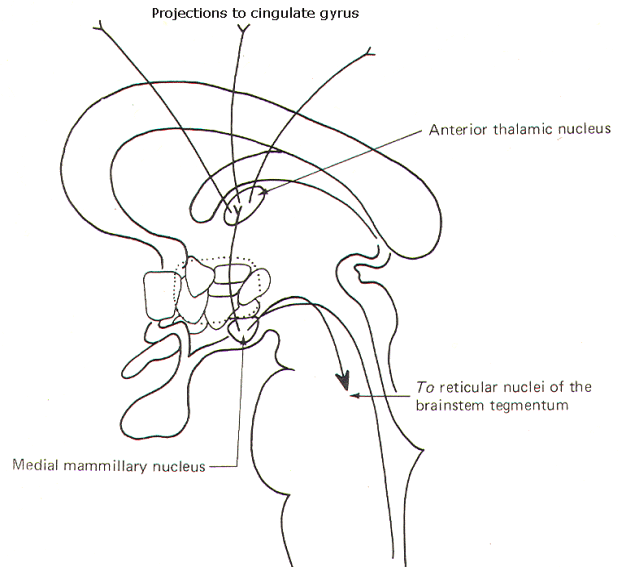 |
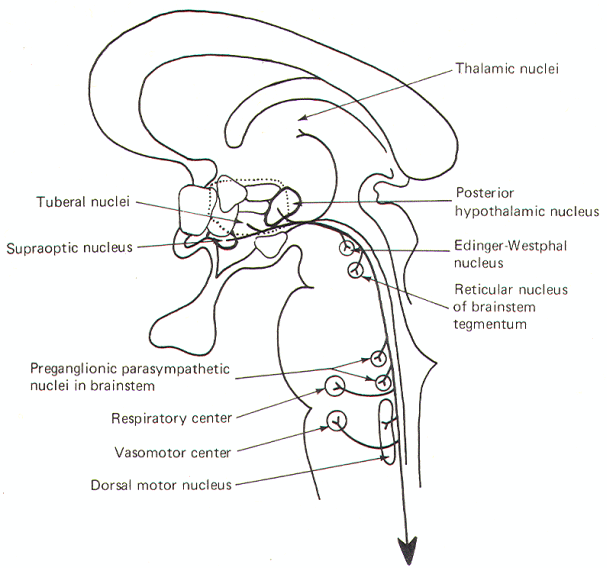 |
Fig-8 |
Fig-9: |
The
periventricular
fibers
represent a large descending fiber system
originating in the supraoptic, posterior
hypothalamic, and tuberal nuclei. While there is a
small ascending component to thalamic nuclei, most
of the fibers descend to synapse in various
parasympathetic brainstem nuclei as well as the
respiratory and vasomotor centers. Some also
terminate in the reticular nuclei of the brainstem
tegmentum. Reticulospinal fibers as well as some
periventricular fibers which don't synapse in the
brainstem, descend into the spinal cord to
influence preganglionic sympathetic and
parasympathetic neurons in the intermediolateral
region (Fig-9).
The
hypothalamohypophyseal
tract
is a group of fibers which run from the
paraventricular and supraoptic nuclei to the
posterior lobe of the pituitary gland. This tract mediates
release of the posterior pituitary hormones, oxytocin, and antidiuretic hormone (ADH). Oxytocin is synthesized in the paraventricular
nucleus and transported through the axons of fibers
projecting to the posterior lobe. ADH is synthesized
in the supraoptic nucleus and similarly transported through the hypothalamohypophyseal tract
to the posterior lobe (Fig-10). The hormones are
stored in the terminal endings of these fibers until
they are released into the circulation.
|
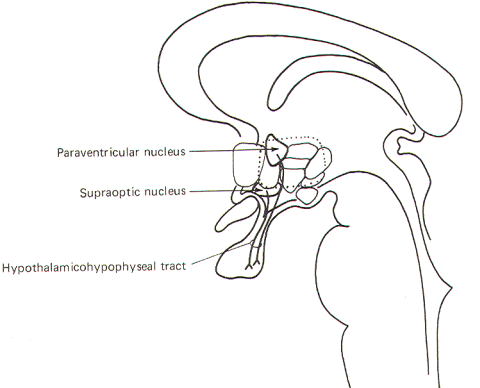 |
|
|
Fig-10 |
|
The Hypothalamus and the Autonomic Nervous System
The
hypothalamus has long been suspected of playing a
role in autonomic nervous system regulation. Most
of the evidence for this is based on the
observation that electrical stimulation of various
areas of the hypothalamus produce autonomic effects.
While there is no clear-cut demarcation line.
stimulation of the
caudal hypothalamus generally produces an increase
in sympathetic activity, while stimulation of the rostral hypothalamus produces parasympathetic
effects. It
is
reasonable to assume that the hypothalamus is not
the sole, or even the
principal, regulator of autonomic activity, While it
can certainly modify autonomic activity via direct
and indirect pathways to preganglionic neurons in
the brain stem and spinal cord, we must also
recognize that the hypothalamus itself receives
input from a wide variety of sources in both the
brain and spinal cord. Thus, while the hypothalamus
can certainly modify autonomic response, the
question of ultimate control is certainly larger and
more complex than can be explained by a model based
on hypothalamic control alone.
The Hypothalamus and Temperature Regulation
Temperature regulation is an important homeostatic
activity which is primarily controlled by the
hypothalamus. If we consider the dangerous effects
of temperature extremes on the body, a center
designed for regulating this variable is of obvious
importance.
Electrical stimulation of the anterior hypothalamus,
particularly the supraoptic area, triggers a
thermolytic response, That is, those activities
which cause the body temperature to drop are set
into operation. Conversely, stimulation of the
posterior hypothalamus, particularly the tuberal
area, triggers a thermogenic response, reflected
both in increased heat conservation and production. Thermolytic responses include cutaneous vasodilation
in order to increase heat loss by radiation,
sweating to increase heat loss by evaporation, and
panting in animals like the dog. Thermogenic
responses include cutaneous vasoconstriction to
prevent heat loss by radiation, shivering to produce
heat by increased muscular activity, cessation of
sweating to reduce heat loss by evaporation, and an
increase in the production and release of thyroxine
in order to increase the metabolic rate.
Thermoreceptors in the hypothalamus are sensitive to
very small changes in the temperature of circulating
blood. Because blood temperature varies closely with
changes in core temperature, the hypothalamus is
continually kept informed of changes in the overall
temperature of the body. Subsequently it can
activate appropriate thermolytic or thermogenic
activities in order to restore body temperature to
normal. The hypothalamus also receives input from
cutaneous thermoreceptors which keep it informed of
changes in the environmental temperature.
Consequently the hypothalamus is continually
informed of both external and internal temperature
changes and is well equipped through neural
activation of appropriate effectors to prevent
temperature fluctuations by regulating body
temperature within very narrow limits.
The Hypothalamus, Thirst, and Control of Body Water
The hypothalamus is well equipped to respond to changes in the
total amount of body water. A poorly localized area of the
hypothalamus called the
"thirst center"
is stimulated by a dry mouth as well as body dehydration,
Projections from the thirst center to the thalamus and then to
the conscious cortex inform
us of
the need for water. This triggers the sensation of
thirst and initiates the conscious desire for water.
The
hypothalamus also takes subconscious steps to
correct dehydration.
Osmoreceptors in the supraoptic nuclei respond to
dehydration (typically associated with increased osmolality in the circulating blood) by increasing
the production and release of antidiuretic hormone (ADH).
This hormone is produced in the supraoptic nucleus
(SON) and transported via the axons of the
hypothalamohypophyseal tract to the posterior
pituitary lobe for temporary storage and ultimate
release into the circulation. Once released, ADH
promotes an increase in total body water by
facilitating water reabsorption in the kidneys so
that more is returned to the blood and less is lost
in the urine. ADH operates by increasing the water
permeability of the distal tubules and collecting
ducts of the nephrons. This causes water to be
osmotically reabsorbed from the less osmotic
glomerular filtrate to the more osmotic
extracellular fluid of the kidney medulla and renal
blood supply.
The Hypothalamus and Appetite
Studies
on animals have confirmed the relationship between
the hypothalamus and appetite. The lateral
hypothalamic nuclei function in part as a "feeding
center." This is based primarily on the observation
that electrical stimulation of this region in the
rat triggers a strong feeding response which is
observed even if the animal has just eaten his fill.
Conversely, the ventromedial nucleus is described as
the "satiety center" because stimulation of this
region stops all feeding activity on the part of the
animal. It is certainly possible that these two
nuclei are neurally related in such a way that each
inhibits the other. In this way, when the lateral
hypothalamic nucleus is directing feeding, it can
also simultaneously inhibit the satiety center, and
vice versa. At present, the system is poorly
understood in humans. If such a mutually exclusive
system exists, however, it is obviously capable of
conscious modification, as we can eat when full and
refrain from eating even when hungry.
The Hypothalamus and the Endocrine System
If, as
it is often said, the pituitary is the master gland
of the endocrine system, it can equally be said that
the hypothalamus is master of the pituitary. It
influences the production and release of hormones
from both the posterior lobe (pars nervosa or neurohypophysis) as well as from the anterior lobe
(pars distalis or adenohypophysis). Unlike the
anterior lobe, which is not derived from neural
tissue, the posterior lobe has an intimate
embryological relationship with the hypothalamus.
Because of this difference, the hypothalamus exerts
its influence in a different manner on each lobe.
Control of the Posterior Lobe The two known posterior pituitary
hormones are oxytocin and antidiuretic hormone, also called
vasopressin. Each is an ~ whose amino acid sequence is well
known. There are no secretory cells in the posterior pituitary,
however, and both hormones are produced in the hypothalamic
nuclei and subsequently transported to the posterior lobe.
Oxytocin
is
probably produced in the paraventricular nucleus (PVN).
Its target tissues include the breast. where it
promotes the letdown of milk, and the uterine
musculature. where it promotes smooth muscle
contractions. It's released in response to several
stimuli. These include mechanical stimulation of the
nipple area by the suckling infant. uterine and
cervical contractions associated with birth. and
psychic factors via poorly understood circuits from
the conscious cortex. The latter is apparent when
the cry of a hungry infant is often a sufficient
stimulus for milk letdown in the lactating mother.
requiring no mechanical stimulation at all.
Antidiuretic hormone
is
produced in the supraoptic nucleus and similarly
transported to the posterior lobe. The stimulus for
its release (stimulation of the thirst center,
dehydration, and increased body fluid osmolality)
have previously been discussed. ADH is also called
vasopressin because of its ability to va
soconstrict blood vessels. Once synthesized, the
hormones are transported to the posterior lobe via
axonal transport through fibers of the
hypothalamohypophyseal tract. Here they are
temporarily stored bound to a protein (neurophysin)
until their release is called for.
Control
of the Anterior Lobe There are no direct nerve fiber
pathways from the hypothalamus to the anterior lobe.
And unlike the posterior lobe. it is rich in
secretory cells. Thus, the hormones of the anterior
lobe are both produced in and released from the
adenohypophysis. The known hormones from the
anterior lobe include: growth hormone (G H),
adrenocorticotrophic hormone (ACTH),
thyroid-stimulating hormone (TSH).
follicle-stimulating hormone (FSH), luteinizing
hormone (LH), luteotropic hormone (L TH), and
melanocyte-stimulating hormone (MSH). Luteinizing
hormone is called
interstitial cell-stimulating hormone
(lCSH)
in the male.
While
these hormones are actually synthesized in the
anterior lobe of the pituitary. the signal for their
release comes from the hypothalamus in the form of
small polypeptides called
releasing factors.
At the
appropriate time a particular releasing factor is
secreted near the capillary network in the median
eminence (Fig-11) by fibers from one or more of
the hypothalamic nuclei. It then diffuses into the
capillaries and travels into the adenohypophysis via
the
hypothalamohypophyseal portal system.
Once in
the anterior lobe. the portal system again gives
rise to a capillary network. The releasing factor
then diffuses out of the capillaries and causes
specific groups of secretory cells to release their
hormone into the capillaries for distribution to the
main circulation. Figure 15-10 illustrates the
various known releasing factors as well as their
hormones and target tissues.
|
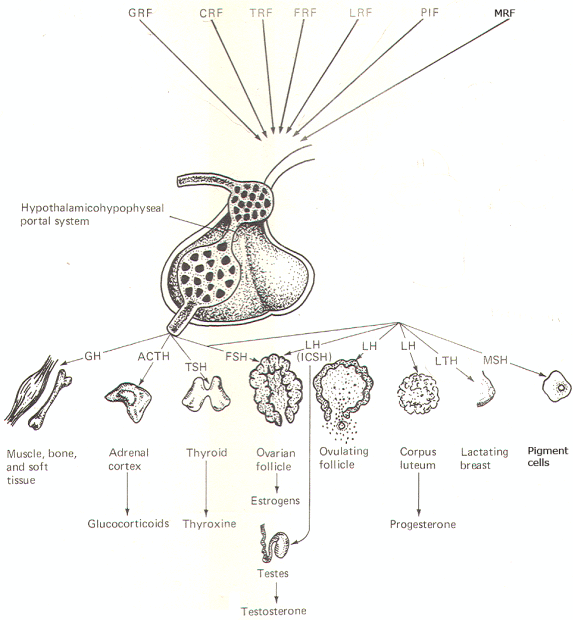 |
|
|
Fig-11 |
|
The Hypothalamus and Emotion: The Limbic System
In addition to its other functions, the hypothalamus also plays
a role in the physical expression of emotion. Parts of the
hypothalamus are closely integrated with the
limbic lobe
of the brain. This lobe. illustrated in Fig-12,
includes the cingulate gyrus, isthmus, and
parahippocampal gyrus and uncus. The limbic lobe
together with the amygdala, hippocampus, olfactory
bulbs and trigone, fornix, and mammillary bodies
comprise the
limbic
system.
In
lower
vertebrates this system is primarily involved with
smell. However in humans, its principal role appears
to be in the arousal of emotion.
The cerebral cortex is associated with the subjective aspects of
"feelings"
and
emotions while the autonomic nervous system promotes
many of the physical expressions associated with
them. It does this through changes in such
activities as heart rate, blood pressure, sweating,
salivation. and gastrointestinal activity. One
theory is that the limbic system ties the
cerebral and autonomic components of emotion together.
We all know that it is possible to worry
enough about something to the point where it
brings on physical symptoms such as stomach upset,
sweating, etc.
Figure-12
illustrates a model for this phenomenon. The
conscious neocortex is reciprocally connected to the
cingulate gyrus. which in turn transmits to the parahippocampal gyrus and uncus of the temporal lobe
via the isthmus. These cortical areas
project to the subcortical hippocampal and amygdaloid nuclei. Fibers projecting from these nuclei pass
through the looping arch of the fornix to the
mammillary nuclei. These, together with other
hypothalamic nuclei. promote autonomic responses
through descending fibers to autonomic nuclei within
the brain stem and spinal cord.
|
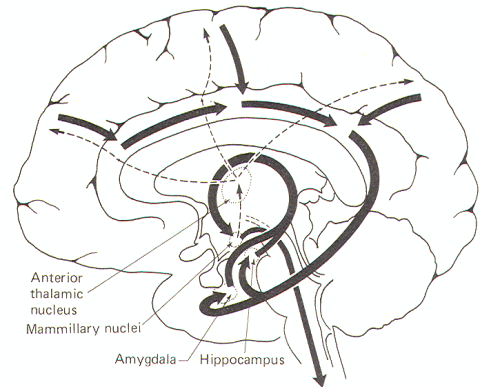 |
|
|
Fig-12 |
|
The system probably works in reverse also. If strong autonomic
activity is going on at a subconscious level, the conscious
cortex often becomes aware of it. This awareness is probably
mediated over mammillothalamic fibers which project to the
anterior nucleus of the thalamus, which then project to the
cingulate gyrus and the conscious cortex. It must be understood
that the pathways described here certainly do not represent the
complete network between the cerebral and autonomic components
of emotion. This is clearly an area about which we know very
little.
|